|
The Quasiturbine team has admiration for Felix Wankel and his successors.
The following comparison is not
intended to discredit their accomplishments,
but to establish the
factual differences with the Quasiturbine technology.
Theory - Quasiturbine versus Wankel
Wankel Drawbacks
STATE OF THE ART: The Wankel engine has
for over 60 years been the dreaming hope of the perfect rotary machine, but
failed to imposed itself for obscured theoretical reasons affecting its
efficiency, and for it internal heavily unbalanced vibrating rotor affecting its
long term robustness. Wankel machine modulate internal chamber volume in two
ways, one being the rotor rotational movement, the other being is eccentric
crank shaft moving the whole rotor from and toward the lateral surface of the
oval stator. Quasiturbine researcher team have shown that these two processes
are not exactly synchronized. While one is attempting compression, the other
process is for part of the cycle, attempting expansion! Referring to piston
engine, this would be somewhat like if the sparkplug would move up as the piston
is compressing upward? This complex intrinsic self-antagonism is destroying
optimum cycles of the Wankel machine, and no simple fix can be imagined… Quite
the end for the Wankel of 60 years of dream about superior rotary machine?
By opposition, the Quasiturbine has no eccentric crankshaft, and the rotor
rotation alone is modulating the chamber volume, while the rotor stay at all
time centered and perfectly balanced. There is no complex self-antagonism volume
effect within the Quasiturbine. Quasiturbine efficiency and robustness has been
demonstrated and recognized by number of different units and sizes.
Today's Wankel engines technology is well
mastered, but the concept does still present major drawbacks. Because hundreds
of experts could not pin point the exact reason for the poor Wankel combustion,
they have "vaguely attributed it without proof" to the elongated shape
(high surface to volume ratio) of the Wankel combustion chamber. The fact is
that even numerical model calculations could not account for the level of
deficient combustion in the Wankel, and it has to be something else... (see
below). Here are
3 important Wankel drawbacks:
-
The Wankel stator bumps not only divide the
chamber into behind and front zones. Just before the TDC (top dead center), the
behind rotor face reduces the chamber volume, while the front rotor face
increases it, which tend to produce a long constant volume pulse near TDC.
Conversely, slightly past the TDC, the front rotor face increases the volume,
while the behind surface reduces it, working against the combustion pressure.
Furthermore, while the crankshaft keep running, the rotor rotation stops near
TDC as the rotor face makes a pure translation movement (on some Wankel design,
not only the rotor stops rotating, but it goes momentarily backward).
-
Square piston (stroke equal to diameter) as the Wankel
produce near TDC in the combustion chamber a modest converging
draft toward the cup, while the QT converging draft is much more important,
and favor better ignition.
-
A complete stroke
ends when the next face come back at that same position. Each Walkel faces are making 3
full strokes per rotor revolution, and there are 3 of them, for a total of 9 full
strokes, or 12 truncated strokes when attempting to make a combustion Walkel
engine. The Quasiturbine fires 4 times with a 4 faces rotor, for a total of 16
jointed and completed strokes per rotor revolution.
-
Low efficiency - Often wrongly attributed to a
slow combustion due to the Wankel rotary elongated chamber geometry (If this
was the case, the Wankel should have been efficient at low rpm where it has
much time for combustion, and inefficient only at higher revolution, which is
not the case). Poor combustion rather results
from the failure of the Wankel concept to meet the Pressure-Volume diagram, due
to an excess volume (positive at intake, negative at relaxation) in the moving
chambers. The increase of pressure level at TDC prevents compensation from intake
to relaxation (This is a theoretical deficiency of the concept which cannot be fixed
by mechanical improvements).
-
Oil leak - The Wankel leaks result from the
fact that the central crankshaft area is part of the oil pan, and a small
quantity of oil tends to flow downward by gravity into the lower chamber.
-
Engine ports overlap - This is a major
limitation of the concept, preventing port design optimization. Because the Wankel attempts to make 4 strokes with a three sides rotor of surface moving
also radially, there is a very large overlap of the intake and exhaust ports,
which imposes to trunk the power stroke somewhat before the bottom dead center
BDC, which results in some lost of efficiency.
In most crankshaft machines, the moving piston
closes the gap with the
piston head (TDC - Top Dead Center) at the most distant point from the
engine center, while in the Wankel (also a crankshaft engine), the moving triangular piston
surface closes the gap (TDC) at the housing area nearest of the engine center!
This means that not only the triangular piston surface moves further inward
during the stroke, but at the same time the housing wall moves outward as the
relaxation progresses! An illogic working behavior, which is not however necessarily a drawback in itself
if the difference in swept volume produces pure rotary forces and if the engine
can extract it? This shows one of the Wankel paradoxal behavior, and invites
everyone to be most careful in understanding engine concepts... Vaguely
attributing combustion deficiency of the Wankel to its elongated combustion
chamber shape
(or its higher surface-to-volume ratio) is a guest or a fast way out, not well
done
science...
Why are not all the combustion chambers
equivalent?
In most engine concepts, the shape of the combustion chamber is not the
dominant combustion factor, but it is rather the closing ability near top dead
center which impact the most the combustion performance. The best chambers do
close near top dead center in such a way that most of the mixture is gathering
into a compact, preferably near spherical volume. Piston closes like 2 proximity
disks, gathering the mixture into a compact volume design, so does the
Quasiturbine in a compact chamber localized within the rotor surface. A
piston having its mixture spread across its two disks geometry at top dead
center has a very poor combustion characteristic. Because of
the Wankel bumped shape, the chamber does not have such a property, as most of
the mixture spreads all along the chamber at top dead center. Furthermore,
because the crankshaft need to be located close to the surface of the triangular
piston (not a Quasiturbine limitation), it is not possible in the Wankel to dig a near spherical chamber within
the rotary piston surface, and the rotary piston surface cavity needs to be
spread along the shape of the chamber.
Square piston (stroke equal to diameter) as
the Wankel
produce near TDC in the combustion chamber a modest converging
draft toward the cup, while the QT converging draft is much more important,
and favor better ignition.
The Wankel explanation attempt based on
centrifugal in-homogeneous mixture (or high gas speed at peripheral) is in
contradiction in rotary engines, with the gas rolling mixing effect between the
two near surfaces moving one in relation to the other. This effect provides a
mixing capability the piston is not able to reach. Poor flame front speed is no
direct argument neither, as the Wankel deficiencies exist at all rpm. This is
not the only Wankel weaknesses, but for all these reasons, it is not appropriate
to associate the Wankel deficiencies to the Quasiturbine concept.
Engine Exhaust Heat Recovery:
By placing a hot Quasiturbine into or around an engine exhaust pipe, and
injecting pressurized hot water (steam keep in the liquid state for better heat
transfer), some heat can be recovered into mechanical energy. Stirling and short
steam circuit Quasiturbine could do similarly!
Wankel Analogy -
A piston with the cylinder head moving down!
In order to help understand why the Wankel poor
combustion results from the failure of its concept to meet the Pressure-Volume diagram due
to an excess volume (positive at intake, negative at relaxation) in the moving
chambers, lets use an analogy with piston (This is a fundamental theoretical
swept volume deficiency of the concept which cannot be fixed
by mechanical improvements). Consider just one of the Wankel rotor surface,
horizontal when at TDC (Top Dead Center) and vertical when at BTC
(Bottom Dead Center). By analogy to a piston, the distance (radius) from the engine
center to this rotor
surface when horizontally (TDC) and then when vertically (BTC) corresponds to the crankshaft drop (stroke) of the "piston
surface equivalent", in relation to the engine center.
In a piston engine,
the "cylinder head" is fix and does not move during the piston stroke,
so that the residual minimum chamber volume at TDC (this excludes the
chamber cut inside the rotor surface) is part of the relaxation volume
during the complete stroke, and does not vary.
Now, observe the residual minimum volume in the Wankel at TDC (which cannot be reduced due to the Wankel concept geometry), and notice its
variation during the strokes as the apex seal move into this minimum
volume area. Contrary to piston, the minimum TDC residual volume is not
all present in the chamber during the stroke, which spoils the
applicability of the Pressure-Volume diagram and its efficiency
criteria.
By analogy to piston, it acts as if the piston head would move
down (taking energy) during relaxation, preventing the complete relaxation
sweep volume to occur, with a corresponding reduction of efficiency... The opposite
volume variation occurs at intake, according to a moving up cylinder
head analogy during compression, which prevents full expected sweep
volume compression to occur. To illustrate how the Wankel excess volume (positive at
intake, negative at relaxation) can affect the combustion relaxation
efficiency,
lets remember that the pressure pulse is in geometric relation to the
volume pulse (faster if considering temperature effect).
The first 50% chamber volume variation doubles the atmospheric absolute pressure to 30 psia,
while the next 25% volume variation brings it to 60 psia; the next 12.5% to 120 psia;
the next 6% to 240 psia ( = 15 bar).
This means that a 6% excess volume could result in a 100 psi pressure
difference near TDC !
Because the Wankel residual chamber volume at TDC (this
excludes the chamber cut inside the rotor surface) cannot be reduced due
to the Wankel concept geometry, its efficiency deficiency cannot be
fixed. On the contrary, if you look at the Quasiturbine residual chamber
volume at TDC, you will notice it can be reduced to zero, which allows
the Quasiturbine to meet the Pressure-Volume diagram efficiency
criteria, just as well as the piston can, and this is a prerequisite for
any better engine concept...
Quasiturbine is Different
The similarities between the Quasiturbine and the Wankel are
like the ones between the planets Earth and Mars,
the closer we look, the fewer
there are!
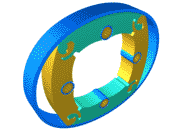
Wankel
Quasiturbine
The Quasiturbine
offers many major improvements over the Wankel, including the following:
-
suppressing the excess chamber volume;
-
suppressing the 3 times 30 degrees dead times;
-
suppressing the need of a rotor-stator synchronization gears;
-
substantially reducing the elongation of the combustion chamber;
-
Allowing full optimization of the ports overlapping;
-
Allowing very high compression ratio, geometrically impossible
in the Wankel.
During 2 shaft (not the rotor) rotations, the Wankel rotor does
rotate 2/3 of a turn and intakes 2 chambers. During the same 2 shaft rotations,
the Quasiturbine rotor will make 2 complete turns and intakes 8 of its chambers.
During 2 shaft rotations, a 4 strokes piston intakes 1 cylinder; for the same
shaft RPM, everything in the Quasiturbine is happening twice as fast as in the
piston and 8 times more frequently. Pneumatic and Steam Quasiturbine operates
without any synchronization flow valve.
Because the Quasiturbine corrects the Wankel theoretical
deficiency, the Quasiturbine does not have the Wankel limitations...
Which allows an equal size Quasiturbine to almost double the power output, while
maintaining optimum efficiency. In addition, because the Quasiturbine does not
have a crankshaft and its concept can accept carriages, its volume pulse
characteristics can be shaped almost at will, including to make a pulse with
rapid rising and falling ramps with a tip duration 15 to 30 times shorter than
the Wankel and piston, which has unique characteristics particularly suitable
for detonation engine.
Typical comparison:
Engine displacement versus the Total engine volume
4 strokes engine type
Unit displacement
Engine volume
Piston
1
15 to 20
Wankel
1
5 to 7
Quasiturbine
1
1.3 to 2
The Quasiturbine is a positive
displacement turbine
with a total displacement almost equal to the engine volume
(Imagine one day, a 3 liters car engine into a
3 liters volume!)
|
The Quasiturbine may look superficially similar to the Wankel engine, but is quite
distinct from it. The Wankel engine has a one rigid triangular rotor
synchronized by gears with the housing, and driven by a crankshaft rotating at 3
times the rotor speed, which moves the rotor faces radially inward and outward.
The Wankel attempts to realize the 4 strokes with a 3-sides rotor, limits
overlapping port optimization, and because of the crankshaft, the Wankel has
near sinusoidal volume pulse characteristics like the piston. The Quasiturbine
has a four-sided articulated rotor, rotating on a circular supporting track with
a shaft rotating at the same speed as the rotor. It has no synchronization gears
and no crankshaft, which allows carriage types to shape "almost at will" the
pressure pulse characteristics for specific needs, including achieving
photo-detonation.
The Wankel engine divides the perimeter in 3 sections while the Quasiturbine
divides it in 4, for a 30% less elongated combustion chamber. The Wankel
geometry further imposes a top dead center residual volume which limits its
compression ratio and prevents compliance with the Pressure-Volume diagram. The
Wankel has three 30 degrees dead time per rotor rotation, while the
Quasiturbine has none which allows continuous combustion by flame transfer, and
can be driven by compressed air or steam without synchronized valve (also by
liquid as hydraulic motor or pump). During rotation, the Wankel apex seals
intercept the housing contour at variable angles from -60 to +60 degrees, while
the Quasiturbine contour seals are almost perpendicular to the housing at all
time. While the Wankel engine requires dual (or more) out of phase rotors for
vibration compensation, the Quasiturbine is suitable for single rotor engine,
because its center of mass is immobile during rotation. While the Wankel shaft
rotates continuously, the rotor does not, as it stops its rotation (even
reverses) near top dead center, an important rotor angular velocity modulation
generating strong internal stresses not present in the Quasiturbine.
Quasiturbine Rotor Dynamic
The eccentric crankshaft machines reach their maximum
and minimum mechanical extension in synchronization with the pressure
strokes, while in the Quasiturbine, the rotor reaches it maximum and
minimum extension at half-stroke, producing a smooth kinetic transition
near Top and Bottom pressure Dead Center. Le piston atteint ses positions
extrêmes en coïncidence avec le début et la fin des cycles de pression, et
comme lui, le vilebrequin du Wankel impose aussi cette synchronisation,
qui favorise un cognement du rotor sur le stator près des points haut et
bas. Dans la Quasiturbine, les positions extrêmes du rotor correspondent à
l’élongation en losange, alors que les cycles de pressions débutent et
terminent en configuration carrée, ce qui crée une situation
particulièrement heureuse pour la continuité du mouvement de rotation et
le balancement des efforts internes sur le rotor lors du passage (sans
cognement) en configuration carrée, ce qui accentue la compatibilité avec
la photo-détonation.
Une fois la déformation du rotor lancée depuis une élongation losange sur
un axe vers une élongation sur un autre axe, le système des 4 pales
présente une inertie qui assure la continuité (sans cognement) de la
déformation lors du passage à la configuration carrée, là où sont les
principales et violentes perturbations de pression. Notons que cette
inertie de la déformation est freinée par l’action des joints de contour
sur les parois internes du stator dans la région éloignée du centre, mais
que l’effet de rappelle dynamique dû à la pression interne dans les
chambres vient aider ce freinage, voir même le dominer à certain régime.
Quasiturbine-Wankel Differences
The Wankel engine uses a rigid three faces rotor with a crankshaft.
The Quasiturbine uses a deformable four face rotor without a crankshaft.
The Wankel engine shaft turns at three times its rotor RPM.
The Quasiturbine rotor and main shaft turns at the same speed.
The Wankel engine fires only once per shaft (not rotor) revolution (which
means three times per rotor revolution).
The Quasiturbine fires four times per main shaft revolution,
producing a strong and exceptional torque continuity.
The Wankel compression and combustion stroke each
last 120 degree of rotor (not shaft) rotation, of which only 90 degrees is
effective (no chamber volume variation in the first 30 degrees of
compression and in the last 30 degrees of combustion). Exhaust and intake
strokes share together 120 degree of rotation in an excessive overlapping
(they are truncated strokes!). In term of time management, the Wankel is
even worst than the piston.
All Quasiturbine strokes are of equal 90 degrees rotor rotation (not
necessarily duration), with
useful volume variation (like piston) at all angles and without
undesired overlapping.
The Wankel fires 3 times per rotor (not shaft)
revolution for a total of 12 strokes, but because its power strokes are
not jointed (three 30 degrees dead times) and overlapped, they are
penalized 90 / 360 and count for an effective total of 9 strokes per rotor
revolution (even less when considering the effect of excessive
exhaust-intake overlap). Said otherwise, a complete stroke
ends when the next face come back at that same position. Each Walkel faces are making 3
full strokes per rotor revolution, and there are 3 of them, for a total of 9 full
strokes, or 12 truncated strokes when attempting to make a combustion Walkel
engine.
The Quasiturbine fires 4 times with a 4 faces rotor, for a total of 16
jointed and completed strokes per rotor revolution.
The Wankel intake stroke is not making vacuum intake
from the rotor face being at TDC (Top dead center), but beginning later
when the tailing chamber seal closes the exhaust port (stroke truncated at
the beginning), and it does end intaking when the intake port is out,
which occurs before the rotor face gets to BDC (stroke also truncated at the
end). Similarly, the exhaust strokes do not pressured-expulse the gases
until the rotor face becomes at TDC, but stops earlier when the leading
chamber seal gets to the intake port (truncated stroke also both at the
beginning and at the end). Considering that the excessive overlap is not a
productive stroke (furthermore detrimental by permitting some intake
mixture to go straight unburnt to the exhaust), one could alternatively
say that in one Wankel rotor rotation, there is a missing 30 + 15 + 15 +
30 stroke degrees, reducing the 4 full strokes by at least 90 / 360 per
revolution (9 instead of 12).
The Quasiturbine has 16 full strokes per revolution with no
overlapping, and none of these Wankel limitations applies.
In the Wankel, 2/3 of the work is produced by piston like radial crankshaft force,
while 1/3 of the work is done by pure rotational (tangential) force, which the crankshaft is not
optimized to harvest (and for which a synchronization casing gear is needed).
In the Quasiturbine,
100% of the work comes from tangential forces and movement, which the
tangential differential harvests correctly.
The Wankel residual geometric Vmin is substantial and
3/4 of this residual Vmin is swept during the
stroke. Vmin is not constant and the engine does not meet the Pressure -
Volume efficiency diagram.
In the Quasiturbine, the Vmin is potentially zero,
a zero Vmin volume is swept without effect during the stroke. Quasiturbine
meets the Pressure - Volume efficiency diagram.
The Wankel excessive engine ports overlap imposes to
trunk the power stroke somewhat before the bottom dead center BDC, which
results in some lost of efficiency.
In the Quasiturbine, the power stroke extends until its is fully
completed.
When the Wankel engine rotor goes from one TDC (top dead center) to the next, the torque
increases to a maximum value and starts decreasing right away (progressive).
The torque generated by the Quasiturbine (accentuated on AC type) gets
toward a
plateau, and holds this maximum for a longer arc before decreasing, producing a
better overall mechanical energy conversion rate.
The Wankel engine has a dead time. A complete rotor (not shaft) revolution
is composed of three relaxation strokes of 90 degrees each, separated by a 30
degrees rotor dead time.
The Quasiturbine strokes are consecutive, with no dead time, allowing
continuous combustion by flame transfer.
The Wankel engine has a negative excess volume during its
relaxation stroke, which lowers efficiency, and is responsible for some unburnt hydrocarbons.
The increase of pressure level at TDC prevents compensation from intake
to relaxation.
The Quasiturbine has no excessive volume during expansion, for maximum
efficiency, and minimum HC unburnt.
Due to its large residual chamber volume near the TDC, the Wankel cannot
easily be operated in Diesel mode.
The Quasiturbine has no compression ratio limitation and can run in diesel mode.
The Wankel contour seals do
two things: sealing the chambers, and providing some rotor orientation forces
(after some wear of the synchronization gears).
For this reason the seals must have a near absolute positioning which limits
the engine lifetime.
The Quasiturbine rotor orientation forces come from the four carriages (AC
with carriages) or from the cylindrical pads (SC without carriage), but not
necessarily
from the contour seals themselves. In the Quasiturbine, the seals' role can
be only dedicated to sealing the chambers, and they are differential in
positioning, which increases the engine lifetime.
The center of mass of the Wankel triangular piston is moving in circle
with the crank, and this whole triangular mass tends to bang the seals against the
housing, requiring the protection of a housing synchronization gear.
The Quasiturbine has no crankshaft, and its rotor center of mass is
immobile at the center during rotation. Never the Quasiturbine seals need to
oppose and constraint the whole rotor mass, the only force required being the
one to transform a square into lozenge and back to square. This is orders of
magnitude less stress on seals than the Wankel rotary piston without its
synchronization gear...
The Wankel engine cannot operate in continuous combustion. While a full expansion
stroke occurs (rotor revolution of 90 degrees), intake mixture compression is
only partially initiated and not yet ready to be lighted (an additional 30
degrees rotor rotation is required as a dead time).
Quasiturbine mixture is completely compressed and ready to fire at
the end of each expansion stroke, making possible a flame transfer for
continuous combustion.
Due to its one single firing per shaft revolution, and the dead time, the
Wankel engine needs a flywheel.
The Quasiturbine needs no flywheel, and consequently has faster
acceleration.
The Wankel's triangular rotor is an internal engine part.
The Quasiturbine four "pivoting blades" have only one side internal since
the shaft sides are in contact with the external environment, and the area is
available for external blades cooling.
The Wankel engine rotor movement must be synchronized by a gear set to
follow the stator profile.
The Quasiturbine does not require any such a synchronization, and
consequently is much easier to build (Allowing even for strong plastic
(ABS) units for moderate temperature applications).
The triangular rotary piston of the Wankel contains the crankshaft and it
is not possible to deeply dig a compact chamber, making the combustion chamber
at top dead center of the Wankel elongated and thin, and the relative contour wall
and piston movements dragging the mixture into an unwanted rolling movement in
a referential moving with the chamber, turning down (squelching) the
combustion at the chamber extremes.
The Quasiturbine chamber is located in a tangential median cut in the
rotary blade filler tip such that at top dead center, it is squeezed between
the 2 carriage rollers and the Saint-Hilaire profile on which the sparkplug is
located, all such to contain over 80% of the gaseous mixture in a rounded
corners cube like shape.
The Wankel needs 2 sparkplugs because of the gas rolling effect and the
thin flat combustion chamber shape.
The Quasiturbine deep semi-circular or semi-spherical combustion chamber
attenuates the combustion gas rolling effect, and consequently, no squelching
occurs behind the chamber, and one sparkplug is sufficient.
The Wankel engine must make use of 3 seals at the triangle peaks
(Apex), which meets the housing wall with a variable angle on both sides of the
perpendicular (-60 degrees to +60 degrees).
Since the Quasiturbine seals are seated on rocking carriers, they are
perpendicular to the engine wall at all time, preventing the
critical leakproof problem.
The Wankel engine radial seals are at equidistant and fix distances.
The Quasiturbine (AC model type) seals are at variable angular and linear distances,
giving relative geometric enhancement to intake, compression, and gas
expansion.
The Wankel engine is a "rotating piston engine" that is subject to a
constant circular vibration.
The Quasiturbine has a fixed center of gravity during rotation, and is a
true zero vibration engine (like the turbine), since any weight movement is
exactly compensated by symmetric mirror movement through the center. (Be
careful not to confuse vibration with unidirectional counter-torque impulses).
The center of the Wankel engine is part of the oil pan, and contains the
mandatory main crankshaft.
In the Quasiturbine, there is no crankshaft, and the central main shaft is optional. The center of the
Quasiturbine can be empty, and available for electrical components, fan
blades, or other devices.
The Wankel engine has limited port access on lateral covers, due to the
presence of the oil pan.
The Quasiturbine is much more accessible, which allows sealed ports for
high pressure compressor, and efficient continuous combustion transfer between
chambers.
In the Wankel engine, the oil pan is also mandatory for crankshaft, bearings,
gears lubrication and thermalization.
In the Quasiturbine, oil is not a thermal cooling agent, and consequently
oil is only required at seal friction interface. The use of ceramic or
high-tech seals can make the Quasiturbine an oil free engine (Thermalization
being done by the contact of the carrier wheels).
The Wankel engine crankshaft is taking a considerable sideways pressure
load.
Since there is no pressure load imposed on the absent crankshaft of the
Quasiturbine, and since its compressed volume (AC model type) nears and breaks away linearly
from its point of maximum compression, Quasiturbine operation can be made
possible in "photo detonation" mode.
Since the main Wankel engine shaft rotates at three times its rotor speed,
it is more suitable for high RPM end uses.
The Quasiturbine main shaft (rotating at the same speed as its rotor) is
more appropriate for lower revolution uses (e.g. airplane propeller at
only 2000 RPM, generator, transportation, or to reduce gearbox ratio in
current applications).
Since the main Wankel engine shaft rotates at three times its rotor
speed, it is less suitable for low RPM compressors or pumps.
Quasiturbine is suitable for both low RPM compressors and pumps. For the
same engine dimensions and shaft RPM, the Quasiturbine presents a substantial
near 50% power density improvement (and more for power to weight ratio) in its
operational range.
The Wankel has a faster raising pressure pulse compare to the piston engine,
but shows a high pressure plateau near the top dead center, due to the slow
rotor horizontal translation by the crankshaft at that point.
The Quasiturbine (AC model type) has a similar fast rising pressure pulse, but no high
pressure plateau, which makes it thermally more efficient, and more suitable
for photo-detonation, diesel and hydrogen mode.
Due to its geometry, the Wankel exhaust and intake ports overlap extensively,
opening much before the expansion stroke is over, and closing much after the
intake stroke has begun.
The Quasiturbine does not impose such a wide overlap detriment to
efficiency.
The Wankel engine success for direct hydrogen combustion comes from
its intake and combustion stratification.
The Quasiturbine engine offers the same hydrogen advantage, without the
loss of efficiency. Furthermore, the Quasiturbine's rotor offers one extra
insulation chamber (4 instead of 3) to hydrogen intake.
The Wankel engine stator profile is an optimum, which can be generated by
simple geometric envelope.
The Quasiturbine "SAINT-HILAIRE stator confinement profile" cannot be
generated by geometric envelop technique, and eccentricity can still be
accentuated for additional performance.
Square piston (stroke equal to diameter) as the Wankel
produce near TDC in the combustion chamber a modest converging draft
toward the cup.
The Quasiturbine converging draft is much more important, and favor
better ignition.
More Technical
Quasiturbine differences with
the Wankel
|
|